Post-pandemic tourism rushes ahead, causing discomfort in and around touristic attractions amid the summer heat. Elisabetta Povoledo covers for the New York Times the latest frustrations experienced by those hoping to visit Rome’s Pantheon, which now requires a ticket issued by the Italian Culture Ministry.
Visitors are frustrated by the symbolic fee (the place is worth the 5-euro price) and by the confusion on how to get it: the online purchase is complex, and the alternative is not better than the typical high-season waiting in line.
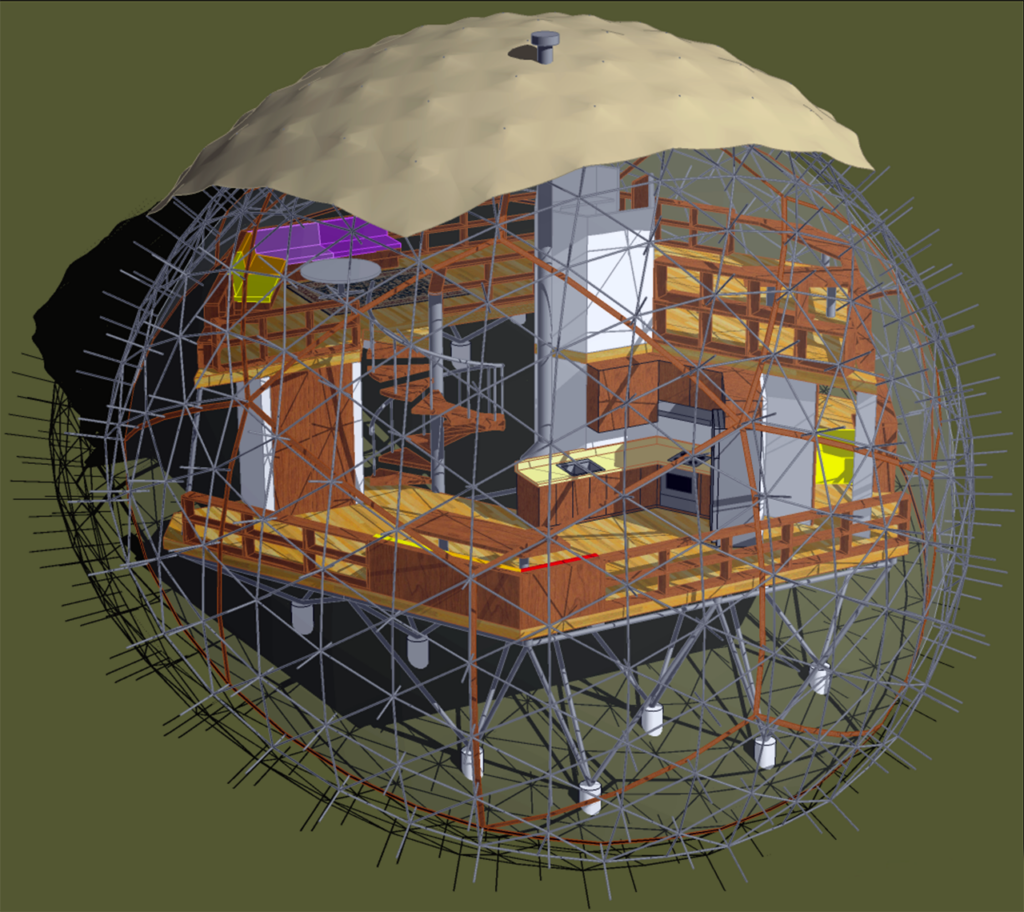
But the place is worth the hassle: the 2000-year-old monument, with a massive domed ceiling built with Roman concrete with an oculus at its center, was the world’s largest for 1,300 years, and it remains the largest unsupported dome in the world.
Arches and domes: a Roman obsession
How did Emperor Hadrian’s appointed engineers do it? Romans realized the structural importance of arches and domes early on and turned such self-sustaining shapes into the critical building block of their civilization, used extensively in buildings and all sorts of infrastructures, from bridges and aqueducts to markets, baths, stadiums, or public latrines.
Unlike previous civilizations across the Mediterranean, Romans understood that their clinging to power would be forever related to their ability to build structures that could withstand the burden of time, extending their utilitarian mighty for centuries.
Today, their engineering rule is still used and remains the best way to share the force of stress across the surface of a given structure in a balanced way. Like an egg that isn’t cracked, no force will collapse an arch or dome that didn’t experience structural damage.
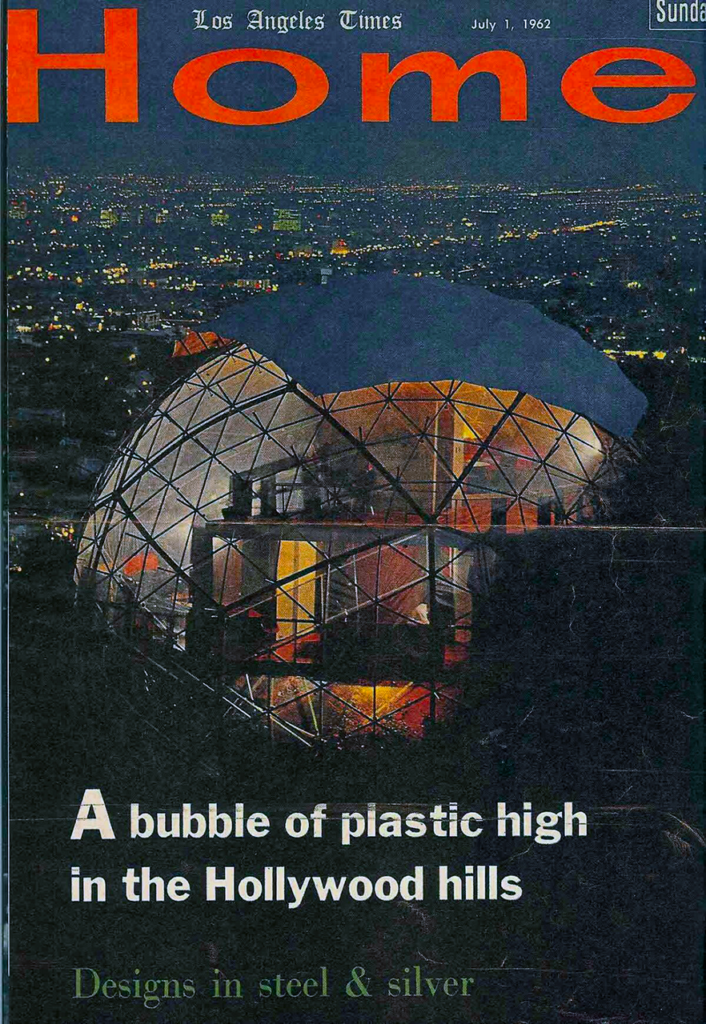
Construction techniques already favored by the Romans (like their arches and domes for thousands of bridges, aqueducts, and buildings that still stand across Europe and the Mediterranean basin) were only surpassed when the Industrial Revolution helped develop materials with bigger structural strength and much higher precision than stone, brick and mortar, ancient concrete, wood, or a combination of them.
Human structures as efficient as cell membranes
Already in the 20th century, the chief engineer of the German optical company Carl Zeiss, Walther Bauersfeld, designed a hemispherical thin-shell structure composed of a convex polyhedron (a solid figure with many plane faces) made from triangles that keep their symmetry.
Bauersfeld used a shape so efficient and scalable that it is ubiquitous among spherical viruses. Not surprisingly, the walls beneath the exterior membrane of complex cells (those with a nucleus, or eukaryotes, like the ones integrating animals, plants, or fungi, are made of proteins) are assembled using triangles made by the intersection of spectrin molecules.
Such scaffolding (or cytoskeleton) is the structure that helps cells maintain their shape and internal organization when they move, composed of a dynamic network of interlinking protein filaments.
Twenty years after Walther Bauersfeld’s construction of a planetarium projector for Zeiss, American architect, and futurist Richard Buckminster Fuller began experimenting with self-reinforcing structures capable of keeping the highest strength with the fewest amount of material; based on a network of circles, geodesic domes sustained themselves through a network of triangles that created an evenly distributed tension throughout a system of nodes interconnected with bars or struts of different materials.
Can we apply the “mechanics of living systems” to housing?
Enamored by a concept that allowed lightweight systems with a high degree of structural integrity and strength, Fuller would soon be associated with geodesic domes, dreaming of geodesic layers for gardens, buildings, or even cities. A polymath from a young age, Fuller had the intuition that the tension-compression equilibrium giving distributed strength to geodesic domes also held key patterns found in the mechanics of living. Today, experts in cellular biophysics study how subcellular structures use the tensegrity principle to keep their shape and stability in a micro world that would fall into chaos otherwise:
“For example, the cell’s surface membrane and supporting cortical cytoskeleton, which are most easily studied in the red blood cell, form a prestressed geodesic (triangulated) structure that gains its mechanical stability by incorporating rigid actin protofilaments that are held in place by surrounding spectrin molecules that act like tensed springs or cables suspended from the overlying lipid bilayer (Vera et al. 2005). Nuclear lamins and interconnected nuclear pore complexes form another type of tensed geodesic lattice that withstands the expansion force of condensed chromatin at the center of the cell (Maniotis et al. 1997, Ingber 2003). A mechanical force balance between compressed microtubules and a tensed network of chromosomes and nuclear scaffolds also appears to stabilize the mitotic spindle (Pickett-Heaps et al. 1997), and this also may potentially explain the ‘coherence’ or mechanical coupling of chromatin structures that has been recently observed over large distances in the living interphase nucleus (Zidovska et al. 2013).”
Tensegrity, cellular biophysics, and the mechanics of living systems, Donald E. Ingber, Ning Wang, and Dimitrije Stamenović, Reports on Progress in Physics, Apr 2014
Like cellular structures and sub-structures, human shelters need to withstand extreme conditions that threaten the shape and integrity of human habitation. Geodesic domes balance mechanical stress by design, with nodes pushing against each other and bonding thanks to gravitational force.
Unlike human domes, the spectrin lattice allows the cell to be flexible: in cells, the triangles formed by the spectrin network underneath the cell membrane are connected at each intersection with actin (a multifunctional protein). Given that the stress isn’t localized but shared by the whole shell, no impact or stress affects the structure to the point of causing rupture.
More volume space, less surface
Cardiac muscle cells are an extreme example of the impressive performance of spectrin lattice structures in eukaryotic cell types. Cardiac muscle cells deal with constant stress due to impact and blood pressure; they evolved to protect the cells’ integrity in the capillaries and veins; without these tiny geodesic structures under the membrane of eukaryotic cells, the complex life of vertebrates wouldn’t have been possible. Red blood cells are rushed around our vascular system to bring oxygen to tissues across the body, the equivalent of taking geodesic domes in flights at supersonic speed.
Like in the walls keeping the integrity of cells, human geodesic domes depend on the balance they achieve once installed; however, geodesic domes are far from self-healing when attacked or rushed around. Could geodesic domes reach such flexibility and strength in the future, thanks to new materials?
Fuller didn’t improve the dome’s strength by observing cells but by looking at the fractal geometry of snowflakes and crystals; he correctly assumed that the structure’s ratio of strength to weight would speed construction and help cope with extreme events such as strong winds, a conclusion ahead of his time in an era in which aerodynamics weren’t taken into account.
After decades in storage, the Smithsonian’s Museum is putting into display Weatherbreak, the first large-scale, self-supporting geodesic dome built in North America. It was conceived and erected in 1950 in a suburb outside Montreal by Jeffrey Lindsay, a designer who studied with Fuller.
After serving in the Royal Canadian Air Force in World War II, Lindsay attended the Chicago Institute of Design; in 1948, he studied architecture with Fuller after following the polymath professor to Black Mountain College, the experimental liberal arts school in North Carolina. In that setup, both Fuller and Lindsay began their fascination for geodesic structures. Fuller had a theory, writes Wendy Moonan for Smithsonian magazine:
“The most stable structural form was not the rectangle but the triangle. He believed that by joining any number of equilateral triangles connected at angles to one another, he could construct a stronger, more stable geodesic dome. And, because a dome encloses the largest volume of interior space with the least amount of surface area, it also saves on material and cost.”
Weatherbreak is born
Fuller and Lindsay enrolled the students during the summer weeks to try constructing the dome, which wasn’t working until they met an innovative sculpture student, Kenneth Snelson. Snelson put together aluminum tubes with wire and elastic bands, exploring the relationship between tension and compression by intuition. Fuller and Lindsey adopted the ideas right away:
“In the summer of 1949, Fuller organized his students into teams dedicated to design, procurement of materials, assembly and manufacture. Eventually, through trial and error, the students succeeded in building a prototype dome with stainless steel tubing and steel wire.”
They quickly realized that the steel wiring was put into tension, it acquired structural force, and when the tension was released, the prototype was easy to disassemble and move to another location.
Fuller’s loose confederation of innovation workshops and offices he had created in 1946, the Fuller Research Foundation, allowed students to keep working near their hometowns. In 1949, Lindsay offered to open one office in Montreal, liaising with the architecture department at McGill University, visiting aluminum’s laboratories in Kingston, Ontario, to explore the possibilities of lighter, sturdier materials capable of withstanding the rules of tensegrity at a big scale:
“By December 1950, having mastered the geometrical equations of the triangles and built the struts accordingly, he was finally able to assemble it with the help of some ski buddies on a field in the suburb of Baie-D’Urfé. When fully constructed, it sat 49 feet wide and 25 feet high and weighed nearly 1500 pounds.”
After visiting the dome, Fuller never fully acknowledged how impressed he had been by Lindsay’s work. He used the concept of tensegrity extensively but never clarified its origin, and when he applied for a patent for geodesics in 1951, Fuller used the drawings and schemes that Lindsay had conceived for Weatherbreak. By 1956, they had ceased collaborating. Later on, Fuller would be in charge of the design and execution of the geodesic dome encasing the American pavilion for Montreal’s Expo 67 (watch the video on our visit to the Montreal Biosphere).
The passion of Bernard Judge
But the life of Lindsay’s Weatherbreak pioneer geodesic dome in North America had another chapter after Fuller and Lindsay parted ways. The Canadian architect moved to Los Angeles to teach in 1954. The entertainment capital welcomed residential experimentation, often on the hills overseeing the city.
When Lindsay met the then architecture student at USC Bernard Judge, an enthusiast of geodesic domes, he offered the then-dismantled dome, which Judge gladly erected to enclose a small house for himself. The project, called Triponent House and Judge’s own living quarters, wanted to become a manifesto of new ways of living light on the land (an economical and attractive living solution using the latest technology), captivating the American public after Life magazine published a photo gallery about the project.
Judge used the term “Triponent” to describe the three elements defining the project: an envelope (the Weatherbreak geodesic dome); the utility core (the building’s plumbing, utilities, kitchen and bathroom appliances, mechanical and electrical systems); and the interior spaces defining life on it.
Conscious of its cultural importance and pioneering character, when he dismantled the house in the 1970s, Judge donated the house to the Smithsonian, which now is assembling it in its museum for the first time.
Bernard Judge would go on to build his experimental home perched over the Hollywood Hills, the AIA award-winning Tree House, but he didn’t give up his idealism. In the early 1970s, Marlon Brando appointed him to create a master plan for a utopian community and sustainable village in Tetiaroa, Tahiti.
An expert’s take on geodesic domes as housing
Judge addressed in 2011 the dreams and contradictions of populating a fragile and then-unpopulated group of islands and lagoon in the South Pacific, in the book Waltzing with Brando, Planning a Paradise in Tahiti.”
As for the fate of geodesic domes, they became a symbol of what’s possible and even needed in human habitation, yet it’s not really wanted by architects, planners, and the public at large. One of the early experts of geodesic domes (and proponent of dome homes early on), the Whole Earth Catalog’s architecture editor and founder of Shelter Publications Lloyd Kahn, described domes as “smart but not wise.”
Our friend Lloyd Kahn (take a look at the Kahn’s Bolinas homestead in this video by Kirsten), wrote decades ago two influential books about domes that inspired such constructions among proponents of the counterculture across the world.
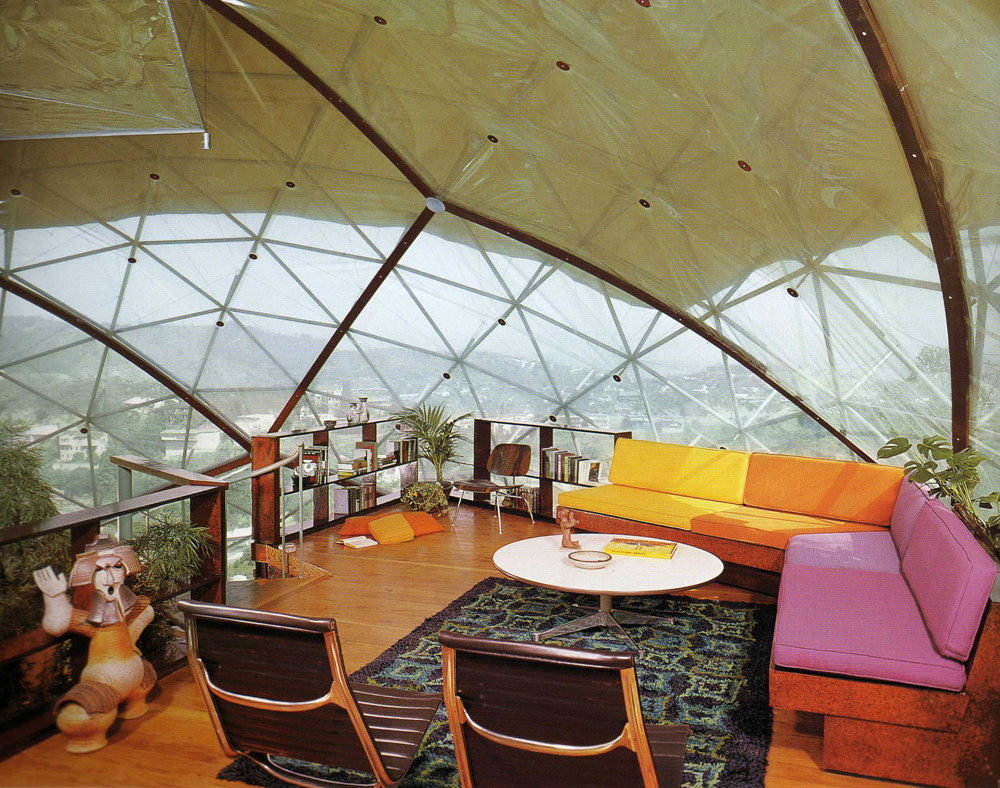
His experience covering the topic for Whole Earth Catalog and the authored Domebook 1 and Domebook 2 make him a trustable source to weigh the pros and cons of such an innovative structure (so innovative, some argue, that it never really took off). According to Lloyd Kahn, using geodesic domes for human habitation means that builders will waste some off-the-shelf building material, which comes in regular shapes; fire escapes can be problematic, whereas wiring is more labor-intensive and expensive.
Kahn also argues that partitioning is difficult in a circular layout and a shape that avoids right angles, which makes it difficult to take advantage of the whole space under the dome and challenges the design of distributions capable of guaranteeing a conventional level of privacy for its occupants.
How to do futurology on pioneer futurologists
Windows and other elements conforming to code can be several times more expensive than conventional ones. Building with natural materials is also challenging since the geodesic structure works best with metals and synthetic materials. According to Kahn, dome builders using cut-board sheathing material found out that the structure was hard to insulate from the elements and tended to deteriorate fast, leading to leaks and requiring artificial insulators.
Moisture can also be challenging on geodesic structures without improved air-exchange systems, similar to those used by airtight dwellings such as passive houses.
Architect James T. Baldwin, a former student of Buckminster Fuller, argues in his book BuckyWorks: Buckminster Fuller’s Ideas for Today, makes the case for efficient, durable, sturdy, no-leak geodesic domes for housing.
Whatever the case, after a recent visit to Berkeley’s Urban Ore, the legendary salvage megastore where one can find anything from housewares, building materials, furniture, clothes, books, industrial tools, vintage music systems, and much much more, having a Bucky Fuller mentality can get anybody with the right attitude in the mood for building things.
Tinkering with post-industrial scraps to innovate in housing should be a more popular proposal nowadays. Domes, for one, are popular candidates among those determined to build disaster-proof homes, writes Christopher Flavelle for the New York Times.
Though at the current pace, it’s more likely that geodesic domes will have a bigger chance to succeed as the building blocks of human outposts beyond Earth than here and now.